
LOCOMOTOR
Y1 SEMESTER 1
These notes were written using learning outcomes as of 2021/2022
We have tried our best to keep all our notes accurate and up to date. However this is the first year these notes have been sent out so we would love your help to improve them. If you notice any mistakes or have any edit suggestions, please send us an email: s1803407@ed.ac.uk
Click on link below to view notes in document format:
Basic mechanics and classification of joints
** plus range of joint movement and stabilising influence of ligaments and tendons ** and relationship between bones and skeletal muscles producing body movements **
BASIC MECHANISMS
Joint = an area where 2 or more bones meet
Components of a synovial joint:
-
Cartilage (covers bone surface, reduces friction)
-
Meniscus = curved cartilage in knees, among other joints
-
-
Synovial membrane (lines the joint capsule, secretes synovial fluid for lubricant)
-
Ligaments (tough, elastic connective tissue bands that surround the joint, giving support and limiting movement)
-
Tendons (connective tissue attaching the muscles that control the joint movement)
-
REMEMBER: a tendon joins muscle to bone, a ligament joins bone to bone
-
-
Bursas (fluid filled sacs that act as cushions sometimes)
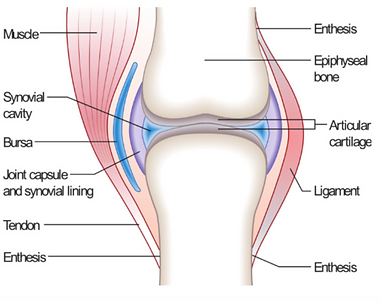
Classification of joints
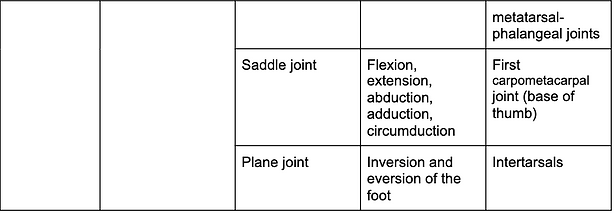
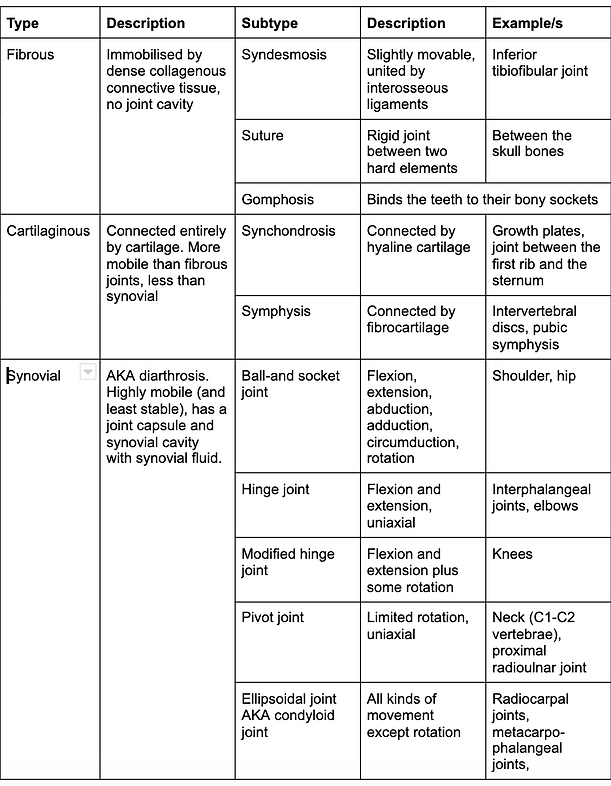
CONNECTIVE TISSUE
Classification of connective tissues, functions, location, composition, histological appearance **
Features of all connective tissue:
-
Cells spread through an ECM
-
Ground substance (colorless, viscous fluid containing glycosaminoglycans and proteoglycans)
-
Fibers (except blood)
-
Collagenous (collagen I)
-
Elastic (elastin and myofibrils)
-
Reticular (collagen III)
-
The ground substance and the fibres create the ECM for the cells to be spread in.
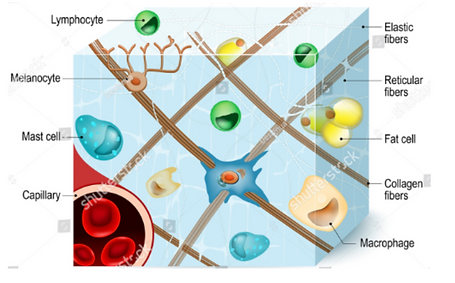
Loose vs dense connective tissue
Loose = more ground surface, fibres loosely organised with large spaces in between
Dense = fibres organised in reinforced bundles
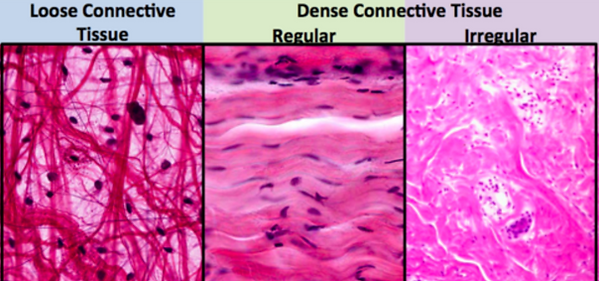
Connective tissue types
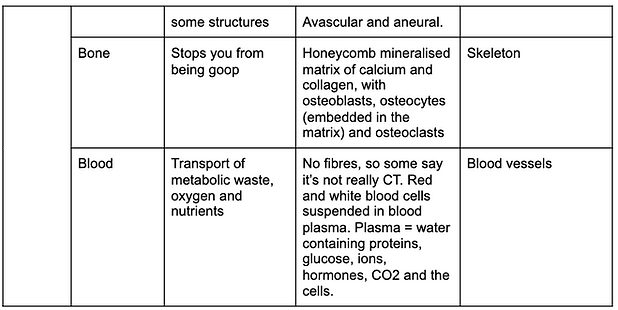
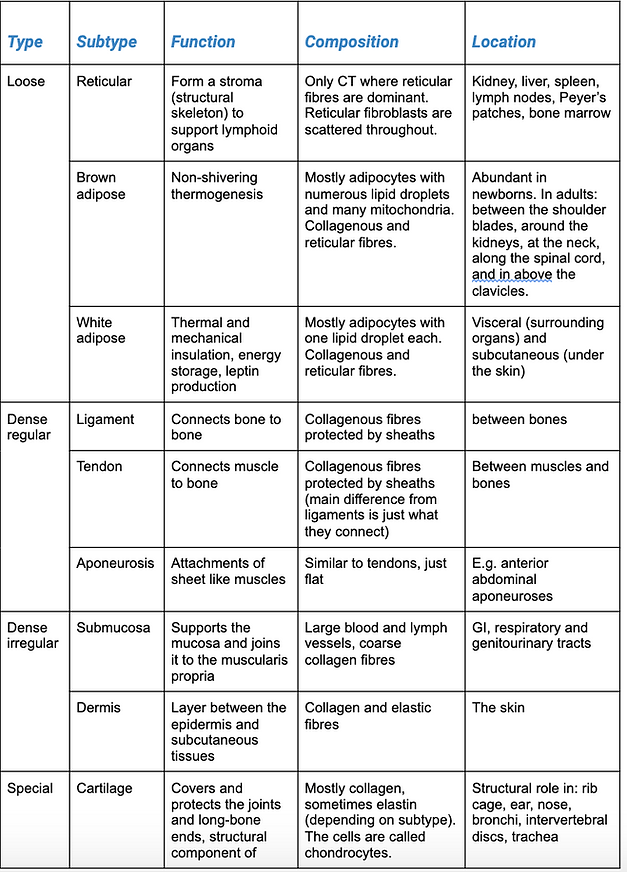
Histological slides of connective tissue
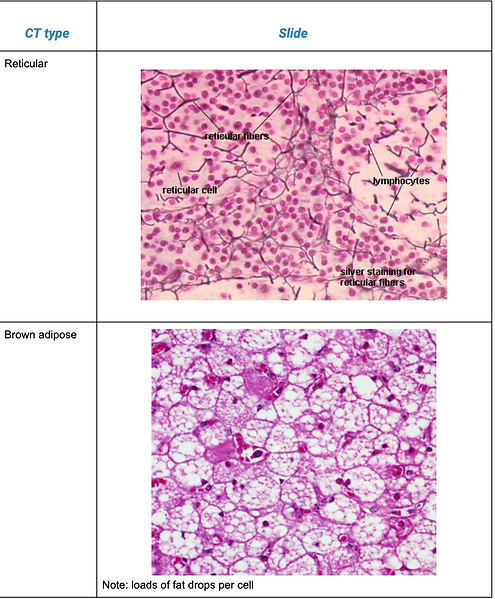

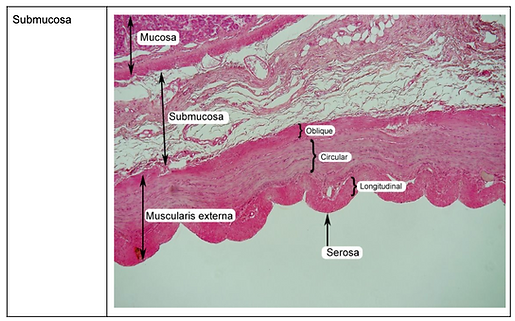

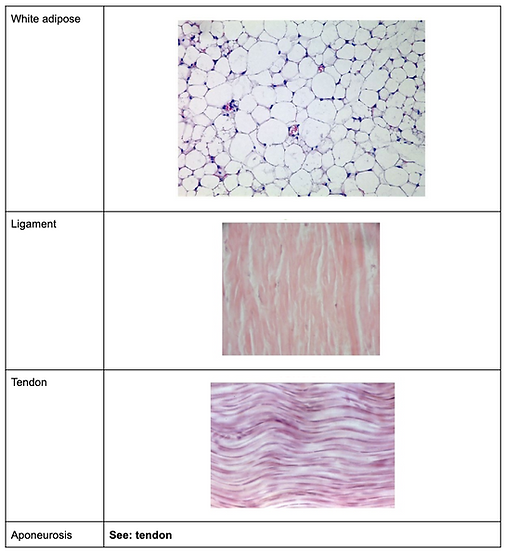
Basic structure and properties of collagen, proteoglycans and elastin **
Collagen
The main structural protein in connective tissue ECMs, and the most abundant protein in the body.
Structure
Amino acids bound together into a triple-stranded collagen helix made up of 3 alpha chains (can either be all the same, or different types of alpha chains).
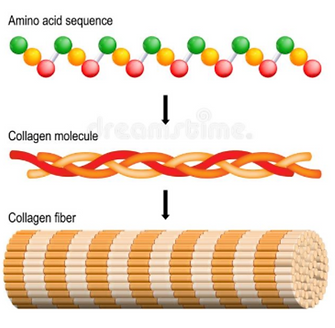
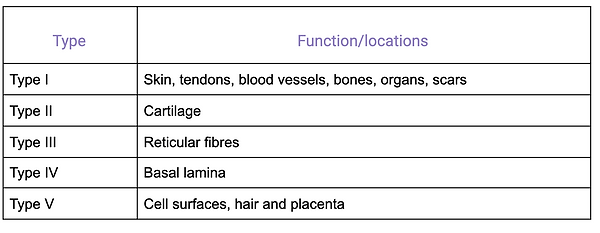
Proteoglycans
A core protein with attached glycosaminoglycan chains present in connective tissue ECMs. They form complexes with each other, with hyaluronan, and with matrix fibres like collagen.
Proteoglycans and type II collagen are the main components of cartilage.
Elastin
Another key protein of connective tissue ECMs.
Elastin and fibrillin come together to make elastic fibres.
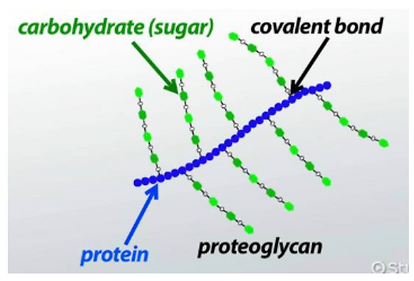
Role of articular cartilage in the joint
Articular cartilage is the cartilage that covers the bones within a joint and allows them to move. As discussed above, it consists of proteoglycans (primarily aggrecan, forming complexes with hyaluronan) and collagens in a matrix. This matrix is produced by cartilage cells called chondrocytes.
Cartilage in joints helps to protect the joint from stresses such as friction, tension and compression. It also has a glycoprotein called lubricin which helps the synovial fluid with joint lubrication and protects the cartilage itself.
Interestingly, the articular cartilage also contains fluid that acts as a synovial fluid reserve - it is mechanically squeezed out during movement to maintain lubrication.
Note that cartilage has very limited repair properties and is difficult to heal.

Properties of chondrocytes and fibroblasts
Chondrocytes
The only cells found in healthy cartilage, isolated in small “lacunae” (little gaps/cavities).
Responsible for the production of the cartilage matrix (mainly collagen and proteoglycans).
Fibroblasts
The most common connective tissue cells in animals.
Responsible for the production of the extracellular matrix (collagen fibres, reticular fibres, elastic fibres, glycosaminoglycans) of all connective tissue that isn’t cartilage (done by chondrocytes) or bone (done by osteoblasts). Also important in wound healing.
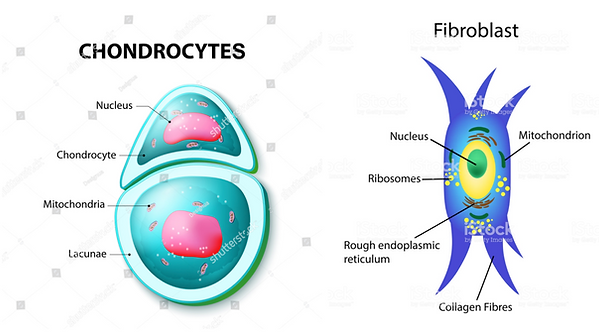
Structure
Covered under “basic structure and properties of collagen, proteoglycans and elastin”
Synthesis and assembly
Mainly occurs in fibroblasts.
-
Genes that encode the relevant alpha peptides are transcribed to mRNA
-
mRNA exits the nucleus
-
mRNA translated at ribosomes; forms pre-pro-peptide
-
Pre-pro-peptide taken into endoplasmic reticulum
In the endoplasmic reticulum
-
N-terminal (start of the peptide chain, named because of the free amine group, recognised by the endoplasmic reticulum) is removed.
-
Peptide now called propeptide
-
-
Hydroxylation of some amino-acids (lysines and prolines)
-
Requires vitamin C, which is why collagen is dysfunctional in scurvy
-
-
Glycosylation (adding of glucose onto the hydroxylated lysines, but not prolines)
-
Twisting of three hydroxylated glycosylated propeptides into a triple helix with unwound ends
-
Known as procollagen
-
-
Procollagen is packaged into a transfer vesicle and taken to the golgi apparatus

Structure, synthesis and assembly of collagen
After the endoplasmic reticulum
-
Oligosaccharides (multiple sugars joined together, e.g. glucose) added in the golgi apparatus
-
Procollagen secreted into the extracellular space
-
Collagen peptidase enzymes remove the unwound ends from the procollagen
-
Now known as tropocollagen
-
Defects here contribute to Ehlers-Danlos Syndrome
-
Step absent for type III collagen
-
-
Lysyl oxidase (enzyme) acts on the lysines and hydroxylysines to produce aldehydes (allow bonding between collagen molecules)
-
Now known as collagen fibril
-
A simpler summary
-
Transcribed in the nucleus and translated in the ribosomes, like all proteins
-
Ends up as pre-pro-collagen
-
-
Post-translational modifications in the endoplasmic reticulum (mostly) and the golgi apparatus (latterly)
-
Ends up as procollagen
-
Problems here are found in both scurvy and Ehlers Danlos syndrome
-
-
Secreted from the cell, undergoes enzymatic modification
-
Ends up as a collagen fibril
-

Composition and functions of synovial fluid
Secreted by the synovial membrane, an ultrafiltrate derived by blood plasma.
Composition
-
Hyaluronic acid (lubricant, secreted by fibroblast-like cells)
-
Lubricin (lubricant, secreted by chondrocytes)
-
Proteinases and collagenases (enzymes)
-
Phagocytes (removes debris from wear-and tear)
Functions
-
Lubrication
-
Shock absorption (it has some weird non-newtonian physics going on that lets it do this)
-
Nutrient and waste transportation (carries O2 and nutrients, removes CO2 and waste from chondrocytes)
-
Molecular sieving (the hyaluronic acid has a secondary function whereby it is forced by the intracapsular pressure to the edges of the synovial membrane, preventing cell migration across the barrier)
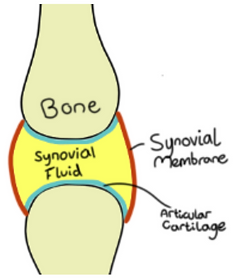
Different types of bone, their structure and function, mineralisation and functions of the various bone cells

Osteoid = the unmineralised, organic component of the bone matrix. Formed by osteoblasts of predominantly type I collagen.
Mineralization = the process by which osteoid becomes filled with calcium phosphate crystals, mediated by osteoblasts
Growth plate**
AKA epiphyseal plate.
This is the hyaline cartilage plate found in the metaphysis of long bones. It provides the cartilage that is ossified (turned into bone) in endochondral ossification.
Endochondral ossification vs intramembranous ossification
Endochondral ossification: the creation of bone from cartilage, essential for the formation and growth of long bones. Occurs at the periosteum (primary center) and the growth plate (secondary center, until the plates fuse).
Periosteum = the connective tissue membrane lining all aspects of the bone except those within joints. Contains fibroblasts and osteoblast progenitor cells (derived from mesenchymal stem cells)
Intramembranous ossification: bone development from fibrous membranes, forming the flat bones of the skull, face, jaw and clavicles. Healing of bone fractures.
Primary endochondral ossification
In utero, the “bone” is a model of what it should be, made of hyaline cartilage. Ossification begins in the diaphysis, with the formation of the periosteum on the outer surface of the bone (made up of osteoblast progenitors).
These osteoblasts in the periosteum develop secrete osteoid (so coming from the outside in) to form the bone collar, which acts as stronger support for new bone.
Chondroblasts in the primary center then secrete alkaline phosphatase (helps with mineralisation) to calcify the bone collar. This is then used as a scaffold for the osteoblasts to form new trabecular/ spongy/cancellous bone.
Osteoclasts play a role here by breaking down some trabecular bone in the middle of the shaft to form the medullary cavity (where the bone marrow is).
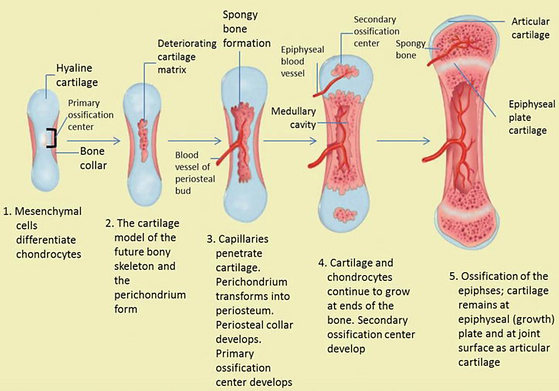
Secondary endochondral ossification
Occurs in the growth plates between the time of birth and the full maturation of the skeleton (at around age 20) when the growth plates fuse.
The secondary ossification center lies above the epiphyseal plate, which is a plate of hyaline cartilage near the ends of the long bones, separating the primary and secondary centers. The process is similar to primary endochondral ossification - the epiphyseal plate forms new cartilage which is replaced by bone via the actions of osteoblasts. This increases long bone length.
Intramembranous ossification
This is the development of the flat bones of the skull/jaw/face/clavicles in utero. It is also how bone fractures are naturally healed (there are mesenchymal stem cells in the medullary cavities beside bone fractures). It does not require cartilage.
-
Initiated by mesenchymal stem cells
-
These replicate to form a dense cluster (nidus) then stop
-
They develop into osteoprogenitor cells, then osteoblasts
-
These osteoblasts create the osteoid and then facilitate its mineralisation
-
The nidus is now bone tissue
-
-
The osteoblasts and osteocytes fuse to form trabeculae
-
During this time there is also osteon/haversian systems formation
-
Osteoblasts connect via cytoplasms to become canaliculi
-
-
-
The trabeculae connect to become woven bone
-
Some mesenchymal stem cells form the periosteum
-
Further ossification occurs at a primary ossification center, much as described in endochondral ossification
-
This creates the bone collar and allows for lamellar bone ossification
-

Diaphysis, metaphysis and epiphysis
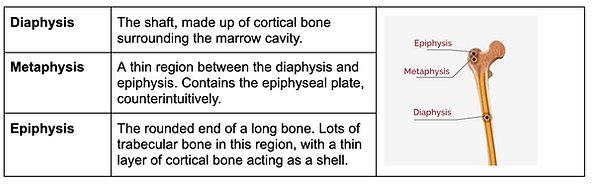
Bone remodelling and calcification **
A lifelong process involving resorption of mature bone tissue and ossification of new bone.
Importance:
-
Reshaping healed fractures
-
Repairing micro-damage
-
Responding to mechanical load requirements
-
Responding to metabolic requirements (e.g. maintaining serum calcium levels)
Resorption
Osteoclasts:
-
Are found on the surfaces of bone being resorbed in Howship’s lacunae
-
Respond to hormones and cytokines then act. They:
-
Secrete acid (H+ ions) to help dissolve the bone
-
Take up dissolved bone minerals and deliver them to nearby capillaries
-
Secrete collagenases (enzymes to degrade organic components of the decalcified matrix)
-
Phagocytose the organic products
-
Ossification in remodelling
Osteoblasts in mature bone:
-
Are found in the periosteum (membrane lining the bony surface) and endosteum (membrane lining the medullary cavity)
-
Secrete osteoid (organic matrix) and mineralise it (produce the enzyme alkaline phosphatase)
-
Become embedded in the new bone as differentiated osteocytes
Osteoblasts and osteoclasts communicate in many ways. For example, osteoblasts signal to osteoclasts through several cell signalling pathways, such as by using RANKL (see: semester 1 week 4). Resorbed bone matrix also releases cytokines that communicate with osteoblasts (e.g. IGF-1, TGF-B). You don’t need to memorise these but it’s important to know the processes are linked and the cells communicate.
Calcium Metabolism
Calcium
-
Function of Calcium:
-
Structural: Ca salt (hydroxyapatite in bones). Without calcium, bones lose density
-
Muscle: needed in contraction cycle
-
Ion channels: sensitive to plasma Ca conc changes, decreases in Ca can cause ion channels to become hyperexcitable or increases in Ca can stop channels from binding or depolarising
-
Protein binding: Ca involved in the clotting cascade
-
Cell Signalling
-
-
Normal total plasma concentration: 2.2-2.6mmol/L
-
Intracellular conc: 7000x lower than plasma
-
allows cell signalling to be very sensitive to changes in Ca concentration
-
-
In plasma, 35-50% bound to proteins, 5-15% in complexes w organic acids and phosphates and the rest of Ca is in ionised form
What organs are central to calcium homeostasis?
-
Bones
-
STORAGE
-
99% stored as Ca salts
-
1% in ECF
-
Exchange: approx 10mmol/day
-
-
TURNOVER
-
Release and sequestration relies on normal bone turnover
-
Osteoclast (OC) resorption
-
PTH: Indirect stimulation as PTH stimulates RANKL from OBs
-
Calcitonin: Direct inhibition of osteoclasts
-
-
-
-
Intestines
-
20mmol per day absorbed
-
25mmol from diet, 15mmol from bile excretion
-
-
Absorbed across brush border of intestinal epithelial cells
-
Dependant on Vit D: Ca binds to calbindin (a vit D dependant protein)
-
-
Regulated by calcitriol (active form of vit D): intestinal absorption is dependent on having adequate vitamin d levels
-
-
Kidneys
-
Filter approximately 250mmol/day and reabsorb 245 for net 5mmol loss
-
Calcitonin inhibits renal reabsorption so there is more Ca lost in the urine; increases renal excretion
-
Parathyroid hormone
-
minor effect: reduces renal excretion
-
major: stimulates vit D to calcitriol (active form) processing
-
-
-
Parathyroid gland
-
Has receptors for serum calcium
-
Parathyroid hormone secreted by chief calls
-
released when low serum Ca is detected
-
Stimulates OB to produce RANKL which activates osteoclasts
-
Also acts on kidneys by increasing renal reabsorption so less is lost from the body and intestines
-
-
-
Thyroid gland
-
Has receptors for serum calcium
-
Produces calcitonin in response high blood calcium levels and functions to stimulate bone deposition by osteoblasts and inhibit osteoclast activity
-
Calcitonin inhibits OC activity
-
Released from parafollicular cells
-
-
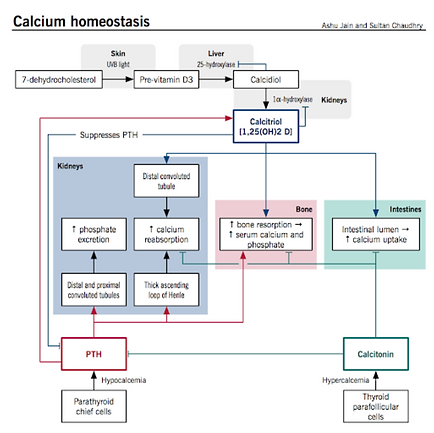
What is the role of vitamin D in calcium metabolism?
-
Used to make calcitriol
-
Obtained from the intestines (lipid soluble) and skin (from UVB generation)
-
After vitamin D is obtained, it is hydroxylated twice
-
First time: In liver via 25hydroxylase to make 25hydroxyvitamin D
-
Second time: in kidneys via 1ahydroxylase to produce calcitriol (1,25dihydroxyvitamin D) ← this is stimulated by either PTH or low serum ca
-
-
Calcitriol stimulates OB to release RANKL to stimulate osteoclast differentiation and to increase GI and renal absorption of Ca
Hyper/hypocalcaemia
-
Hypercalcaemia
-
Detected by thyroid
-
Thyroid releases calcitonin
-
Calcitonin reduces renal uptake so more Ca is lost in urine
-
Calcitonin also inhibits OC activity
-
This all decreases Ca conc
-
-
Hypocalcaemia
-
Detected by parathyroid
-
releases to PTH
-
PTH stimulates OB to produce more interleukins and macrophage colony stimulating receptors AND RANKL
-
This has the effect of activating OC so increasing bone resorption
-
PTH and calcitriol also work together to increase RANKL release
-
PTH causes increasing renal hydroxylation of vit D so more calcitriol
-
This leads to increased intestinal uptake and renal uptake
-
NOTE THAT OC activation route is slowest, intestinal uptake is intermediate and renal uptake is fastest route to combat hypocalcaemia
Effects of age on connective tissues (bone, cartilage) ***

Bones
The most important effect of age on bones is a loss of bone density. This makes bones more fragile, and can result in osteopenia or osteoporosis (see later learning outcomes).
Menopause increases the loss of bone density due to the loss of estrogen, which is normally protective of bones.
Cartilage
Cartilage naturally deteriorates/degrades with age. If there is too much cartilage loss, it leads to a loss of the usual cushioning that cartilage provides to joints, causing osteoarthritis (see later learning outcomes).
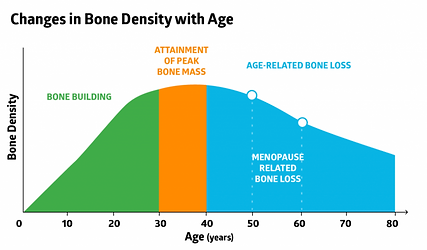
Concept of ‘Form follows Function’ in connective tissues in response to load mechanotransduction ** AND Adaptation of the musculoskeletal system to various forms of mechanical load (static/ dynamic/ trauma) **
Mechanotransduction is the process of converting mechanical stimuli into biochemical activity. This is done via mechanically gated ion channels.
Cartilage acts to absorb mechanical loads on joints. Mechanically gated ion channels in chondrocytes sense these signals to start certain cellular processes such as the synthesis and degradation of the ECM.
High strains (e.g. from impact) cause tissue degradation, apoptosis and decreased synthesis. Lower levels of strain over a long time (e.g. extended bed rest) also cause a loss of synthesis, as does a high static load.
However, oscillating low level loads (i.e. from walking) increase matrix synthesis and thus improve cartilage health. So, static loads and trauma are bad for cartilage; dynamic loads are good.

Core Diseases/ Disorders (presentations incorporated)
Nutritional and inherited diseases of bones and joints (scurvy, Osteogenesis imperfecta, Ehlers-Danlos syndrome, Marfan’s syndrome) **
Scurvy
Pathogenesis
Severe vitamin C deficiency, due either to diet (including eating disorders and complications of addiction, and socioeconomic factors affecting the elderly and the very young) or malabsorption (e.g. IBD).
Humans cannot produce vitamin C, it must come from the diet.
Many of the symptoms of scurvy are due to the fact that ascorbic acid is necessary for collagen synthesis, and collagen is an important structural protein in nearly every tissue (including blood vessels, muscles, skin and bones including teeth).
Presentation
-
Weakness, lethargy
-
Mood problems (irritability, malaise)
-
Joint (arthralgia) and leg pain (myalgia is muscle pain)
-
Skin changes with roughness
-
Swollen, bleeding gums or tooth loss
-
Easy bruising and petechia
-
Poor wound healing
-
Dry eyes and mouth
-
Eventually complications leading to jaundice, edema, oliguria, neuropathy, infectious symptoms and convulsions
Management
Treat the underlying cause if there is one - e.g. induce remission of IBD.
Oral vitamin C supplements and improvement of diet.

Osteogenesis imperfecta
Pathogenesis
AKA “brittle bone disease”. This is a group of genetic disorders that cause bones to break easily, due to a lack of type I collagen.
There are at least 8 types: type 2 is the most severe. Type 1 is the mildest and most common.
It is inherited in an autosomal dominant pattern, but type 2 is always de novo because the patients don’t generally survive to child-bearing age. It’s usually lethal around birth. Type 1 is 60% de novo.
Presentation
-
Multiple fractures
-
Blue sclera
-
Loose joints, poor muscle tone
-
Easy bruising
-
Hearing loss (due to ear deformities)
-
Neurological problems (due to skeletal deformities damaging the CNS)
Management
There is no cure, and management is generally about living a healthy life and avoiding activities that might predispose to a risk of fracture.

Ehlers Danlos syndrome
Pathogenesis
A group of rare inherited connective tissue disorders. There are many genes that can be affected, and the genes involved determine the subtype as well as the disordered tissues.
Presentation
-
Loose, hyperflexible joints
-
Increased rates of sprains, subluxations and dislocations
-
Arthralgia
-
Stretchy, velvety skin
-
Abnormal scar formation
-
Complications
-
Scoliosis
-
Chronic pain
-
Early onset arthritis
-
Cardiovascular events (in those with EDS affecting blood vessels)
-
Management
Supportive - physical therapy to strengthen the muscles that support joints, treatment of complications.
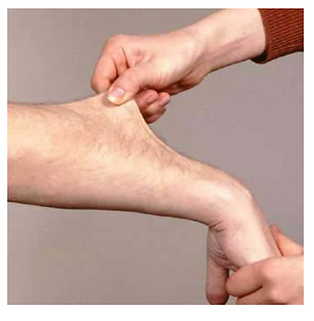
Marfan syndrome
Pathogenesis
Autosomal dominant genetic disorder affecting FBN1 (which makes fibrillin). 25% of cases are de novo.
Presentation
-
Tall and thin patients with long limbs, fingers and toes
-
Pectus excavatum
-
Flexible joints
-
Scoliosis
-
Cardiovascular complications
-
Increased risk of mitral valve prolapse and aortic aneurysm
-
Management
No cure, but beta blockers, calcium channel blockers and ACE inhibitors may be used for prevention of cardiovascular complications and strenuous exercise should be avoided.

Osteoarthritis **
Pathogenesis
Classically considered to be due to “wear and tear”. There is loss of cartilage (which is meant to provide cushioning) and therefore inflammation with remodelling of the adjacent bone.
Presentation
Typically presents with bilateral pain and stiffness that is worse with exercise and improves with rest.
Common in the elderly (equally in men and women).
Osteoarthritis in the hands
Heberden’s (distal interphalangeal joints) and Buchard’s (proximal interphalangeal joints, I remember this because “B” and “P” are both plosive sounds) nodes are bony nodules in the hands that are specific to osteoarthritis.
X ray findings
-
Decreased joint space
-
Subchondral sclerosis (thickening beneath the cartilage)
-
Subchondral cysts
-
Osteophytes at the joint margin
Distribution
Often affects larger weight-bearing joints, such as the hips and knees, as well as the carpometacarpal, proximal interphalangeal joints and distal interphalangeal joints.
Importantly, OA can affect the base of the thumb (first carpo-metacarpal joint) while RA tends not to! This is a good way to tell the two apart sometimes.
Management
All OA patients should be offered help with weight loss and given advice about muscle strengthening and fitness.
First line treatment: paracetamol + topical NSAIDS for knee/hand pain
Joint replacements only once conservative methods have failed.

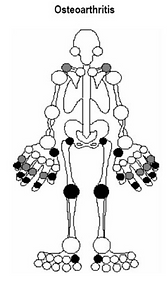
Inflammatory joint diseases, particularly rheumatoid arthritis **
Rheumatoid arthritis
Pathogenesis
An autoimmune condition of chronic systemic inflammation.
Presentation
An autoimmune inflammatory joint disease that tends to present with bilateral morning stiffness and pain that improves with use, with or without systemic upset. Joints can appear swollen.
Seen in adults of all ages, more commonly in women.
X-ray findings
-
Loss of joint space
-
Juxta-articular osteoporosis (thin bone nearby)
-
Periarticular erosions (eroded bone nearby)
-
Subluxations (partial dislocations)
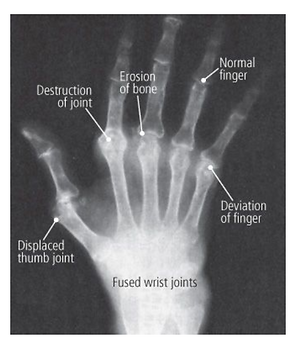
Blood test findings
-
Rheumatoid factor high in 80% of cases (only 50% at the start of the disease) but is also high in other conditions and in 5-10% of healthy people
-
Anti-CCP antibodies more specific to RA, but not present in every case
Distribution
Tends to present first in the small joints in the hands and feet, with larger joints becoming involved later.


Osteoporosis (including ‘silent disease’ with lack of pain) **
Pathogenesis
Osteoporosis is a pathological increased risk of fracture due to low bone density.
Bone density is maintained by a balance between mineral deposition (by osteoblasts) and reabsorption (by osteoclasts). All bone density decreases with age, and the T-score can be used to measure the severity of this. Subclinical bone-thinning is called osteopenia.
People whose bones were thinner to start with are more at risk of falling to a pathological level. This includes women, who tend to present after the menopause because estrogen is protective.
Presentation
Osteoporosis is very common among the elderly, particularly postmenopausal women, but until the first fracture there generally are no symptoms.
Management
Bisphosphonates (e.g. alendronic acid) are the main drug used to treat osteoporosis, as they slow bone resorption. They must be taken on an empty stomach with a full glass of water. After they’re taken, the patient must stand or sit upright for 30 minutes, and wait between 30 minutes and 2 hours before eating or drinking anything else. This is to reduce the risk of esophageal tearing.
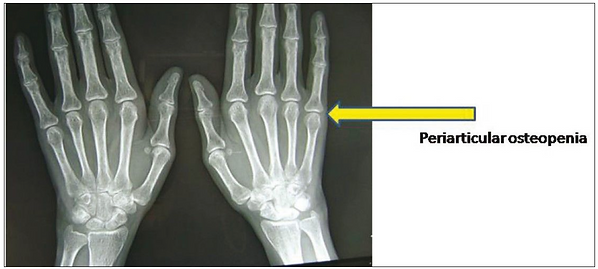

Common conditions of the hand (carpal tunnel syndrome, Dupuytren’s disease, rheumatoid arthritis) **
Carpal tunnel syndrome
Pathogenesis
Caused by compression of the median nerve in the carpal tunnel, eliciting motor and sensory symptoms related to the action of the median nerve. Causes include:
-
Idiopathic
-
Rheumatoid arthritis
-
Pregnancy
-
Lunate fracture (e.g. due to a FOOSH, a fall onto the outstretched hand)
-
Oedema (e.g. in heart failure)
The carpal tunnel
The median nerve is the only nerve to pass through the carpal tunnel.
Presentation
What does the median nerve do again?
Sensory distribution
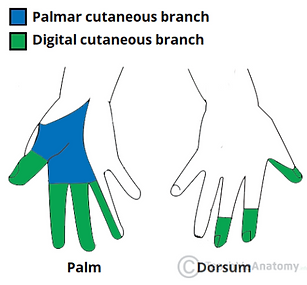
Motor functions
-
Supplies the first 2 lumbricals
-
Supplies the thenar eminence
Symptoms
-
Weakness of abductor pollicis brevis (responsible for abduction of the thumb)
-
Thenar eminence wasting
-
Pins and needles in median nerve sensory distribution when the nerve is tapped (Tinel’s sign)
-
Flexing the wrist (think like praying) for a minute elicits symptoms (Phalen’s sign)
Management
-
Corticosteroid injections
-
Flexor retinaculum division (surgery, to decompress the nerve by giving it more room)
-
Wrist splints at night
Dupuytren’s disease
Pathogenesis
Formation of abnormal connective tissue in the palmar fascia.
Risk factors:
-
White males >50 (Sinatra and Regan both had it, says Wikipedia!)
-
Family history
-
Alcohol excess
-
Smoking
-
Thyroid dysfunction
-
Liver disease
-
Diabetes
-
Epilepsy
-
Hand trauma
Presentation
May first present with hard nodules under the skin of the palm. Eventually, one or more fingers become permanently flexed.
Most commonly affects the ring finger. Typically not painful, but might itch or ache a little.

Management
Steroid injections and physical therapy are first line.
Rheumatoid arthritis
See: “inflammatory joint disease, particularly rheumatoid arthritis”
Osteomalacia **
This is the name for rickets in adults - a bone disease caused by a nutritional deficiency, usually vitamin D.
Pathogenesis
Taking vitamin D deficiency as an example.
-
Lack of vitamin D
-
Less calcium absorption in the gut
-
Lower serum Ca2+
-
Activation of the parathyroid glands and stimulation of PTH production
-
Increased bone resorption (to increase serum calcium)
-
Less bone mineralisation
-
Soft bones :(
Causes of vitamin D deficiency
-
Diet
-
CKD (chronic kidney disease, this is renal osteodystrophy)
-
Malabsorption (e.g. IBD, coeliac)
Presentation
-
Bone pain (diffuse)
-
Muscle weakness
-
Pathological fractures
-
Deformities in children (thick ankles/wrists/knees, bowed legs, soft skull bones)
-
Waddling gait
-
Poor growth in children
Management
Increase vitamin D and calcium intake:
-
Diet
-
Supplements
-
Sunlight
Treat a pathological cause (e.g. induce and maintain remission of IBD, exclude gluten from diet in Coeliac disease)
Treating the complications (e.g. surgery for deformity)
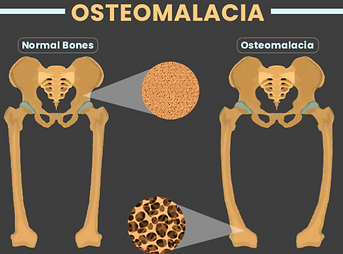
Paget’s disease **
Pathogenesis
Increased and uncontrolled bone turnover → excessive osteoclast activity leads to subsequent increased osteoblast activity. Leads to thickened, sclerotic bones.
Typically affects the skull, spine/pelvis and long bones of the lower limbs.
Risk factors:
-
Age
-
Male sex
-
Family history
-
Being from the Northern Hemisphere
Presentation
-
Bone pain (spine, pelvis, femur)
-
An isolated risk in serum ALP (i.e. calcium and phosphate are normal)
-
Thickened skull vault
-
Deafness, fractures, risk of bone sarcoma and cardiac failure
Management
Bisphosphonates (oral risedronate or IV zoledronate) to slow the bone resorption.
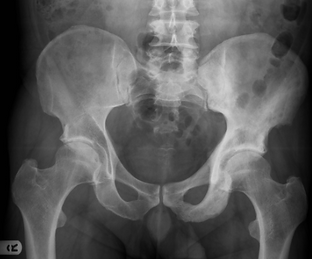
Mechanical trauma to the bones and joints (crush syndrome, compartment syndrome, fat embolism) **
Crush syndrome
AKA traumatic rhabdomyolysis.
Pathogenesis
A crushing injury to the skeletal muscle leads to acute kidney failure. How?
-
Muscles are destroyed due to lack of oxygen
-
Formation of muscle breakdown products:
-
Myoglobin
-
Potassium
-
Phosphorus
-
-
Muscles are reperfused, releasing the products into the bloodstream
-
Something about these products is nephrotoxic
Presentation
Signs and symptoms of AKI following a crush injury:
-
Raised creatinine (but be careful because this is released by injured muscle also)
-
Oliguria
-
Oedema
-
Shortness of breath
-
Confusion, seizures
-
Nausea
Prevention
Don’t release the pressure quickly. First-aiders aren’t meant to release victims of crush injury who have been trapped more than 15 minutes.
Management
There isn’t much to be done to undo it, but rehydrating the patient can prevent kidney failure.
Compartment syndrome
Pathogenesis
Bleeding or swelling in a muscular compartment raises the pressure. This can’t be released as the compartment is enclosed by fascia. This is a limb-threatening condition and an orthopedic emergency.
Presentation
-
Intense, disproportionate pain, particularly on passive stretching
-
Tenderness and a feeling of tightness, tingling or burning
Management
Fasciectomy (surgery to let the swelling happen by opening the compartment).

Fat embolism
Pathogenesis
A lower limb fracture (femur, tibia, pelvis) leads to release or production of fat from the bone marrow. Globules of fat can enter the circulation and travel, lodging as an embolus elsewhere (particularly in the lungs).
Presentation
Hypoxaemia, CNS depression, pulmonary oedema, axillary or subconjunctival petechiae.
Prevention
Immobilisation or internal fixation (surgical) of long bone fractures.
Management
Admit to ICU and monitor GCU and central venous pressure while giving supportive treatment (O2, CPAP or ventilation plus fluid replacement to prevent shock).
Common sports injuries *
Unhappy triad
Damage to:
-
The ACL
-
The MCL
-
A meniscus (medial or lateral)

Iliotibial band syndrome
An overuse injury of the iliotibial band (connective tissue in the lateral thigh). Causes pain and tenderness there, especially just above the lateral knee.
Tennis elbow
AKA lateral epicondylitis. Another overuse injury. A tendinopathy affecting the origins of the extensors of the forearm (which originate at the lateral epicondyle of the humerus). Therefore, symptoms are difficulty fully extending the forearm, and pain:
-
Just below the elbow, laterally
-
When lifting or bending the arm
-
When gripping small objects
-
When twisting the forearm (e.g. to turn a door handle)
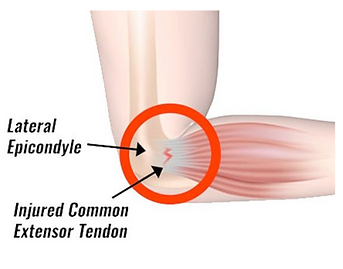
Achilles tendinopathy
Injury to, and pain/stiffness/swelling of, the achilles tendon at the heel/back of the leg. May be associated with crepitus (creaking) when the ankle is moved.
Tearing to the achilles tendon is a sudden intense heel pain associated with swelling and bruising, even potentially with a “snapping” sound. There will be an inability to plantar flex the foot. This is achilles tendon rupture. Surgery is the recommended treatment for young active patients.
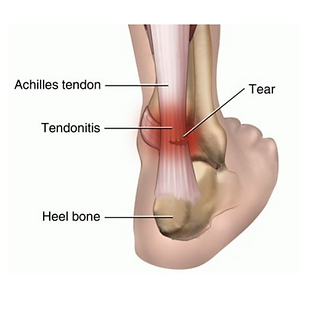

Abnormal development of the growth plate and techniques for treatment *
Epiphyseal fractures
AKA Salter-Harris fractures. Most heal normally without lasting damage. However, sometimes the bone that forms as the fracture heals can cause stunted growth or curving and would need to be surgically removed.
Salter-Harris fractures can also sometimes stimulate growth, leading to uneven limbs. Therefore patients should be followed up regularly for at least a year.
Osteochondroma
The most common non-cancerous bone growth, often found in the legs, shoulder blades or pelvis.
An overgrowth of cartilage and bone near the growth plate. Generally manifests before adolescence.
Most have no symptoms but some can:
-
Limit movement
-
A hard, immobile, painless mass on palpation
-
Adjacent muscle pain
-
Pressure or irritation with vigorous exercise
Doesn’t normally affect bone growth. Complete excision of the growth is curative.
Achondroplasia
A genetic cause of dwarfism with an autosomal dominant inheritance.
80% of cases are de novo, mostly due to a mutation in sperm more common with older fathers.
Inheriting two copies of the gene (e.g. from two parents with achondroplasia) is always fatal for the offspring due to breathing problems.
Caused by a mutation in FGFR3 (fibroblast growth factor receptor 3) leading to overactivity.
In health, the FGF3 protein negatively regulates bone growth at the growth plate (it actually increases chondrocyte development in utero, but postnatally it inhibits it).
If it is always active (due to the mutation), then the bones will be too short.
The dwarfism is described as “disproportionate” or “non-vitruvian” because the limbs will be short while the torso is typically of normal length.
Common paediatric orthopaedic problems *
Slipped upper femoral epiphysis
AKA slipped capital femoral epiphysis. A type of Salter-Harris fracture.
Pathophysiology
A fracture through the growth plate beside the femoral head, leading to the metaphysis slipping out of place. The epiphysis actually remains where it should be because it’s held by ligaments
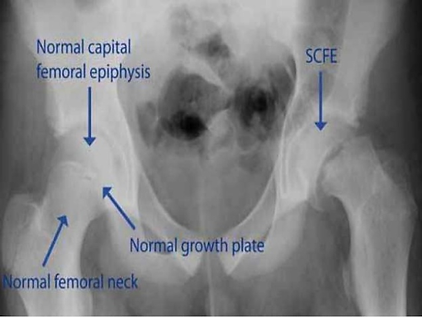
Presentation
Typically in adolescence, particularly young black males, with the major risk factor being childhood obesity.
Symptoms include:
-
Progressive onset groin, thigh or knee pain
-
Painful limp (waddling gait)
-
Limited internal rotation, abduction and flexion of the hip.
Management
Requires surgery, and is an orthopaedic emergency due to the possibility of avascular necrosis (ischaemia of the hip). Involves pinning the femoral head in place (this is without attempting to put it back where it should be, as that can increase the risk of necrosis).
Perthes disease
Pathophysiology
The femoral head is supplied by blood vessels that are easily disrupted
(e.g. by a hip fracture in elderly people with osteoporosis), leading to
ischaemia. The bone then dies. This is called “avascular necrosis” (bone
infarction). In Perthes disease, the blood supply in a child’s hip is
temporarily disrupted and the hip begins to weaken and eventually collapse.
Presentation
Tends to occur in boys aged between 4-10. Signs and symptoms include:
-
Hip pain (progressive over a few weeks)
-
Limp
-
Stiffness and reduced hip movement
-
Can lead to osteoarthritis and premature growth plate fusion
Perthes disease is diagnosed by X ray, which might show widening of joint space and flattening of the femoral head, depending on the stage of the disease.
Management
Very young patients with few changes on X ray will simply be observed. If the blood supply has returned, the bone may heal on its own.
-
Pain can be controlled with anti-inflammatory drugs like ibuprofen.
-
High impact exercise should be avoided, though physical therapy can help to reduce hip stiffness.
If the deformities on X-ray worsen then:
-
Casts and braces might be used for 4-6 weeks to keep the head of the femur from slipping
-
Surgery may be required to realign the bones and allow them to heal

Developmental dysplasia of the hip
AKA congenital hip dysplasia.
Pathophysiology
Due to insufficient covering of the “ball” of the femur by the “socket” of the acetabulum. The cause is unknown, though there is a hereditary component and breech births (baby the wrong way round!) increase the risk as the femur can get displaced.
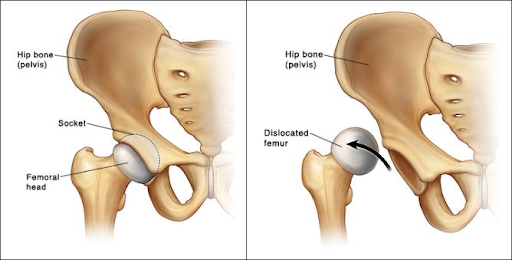
Presentation
Symptoms don’t tend to present until after a year, but the condition can be screened for in newborn exams and 6-month checks with maneuvers involving moving the hips.
Asymmetrical gluteal folds and limb-length inequality are also possible signs.
Management
Careful monitoring to delay the onset of arthritis using harnesses and casts. If the condition is found late, then surgery may be required.
Osgood-Schlatter’s Disease
Pathophysiology
Inflammation of the patellar ligament (holds the kneecap in place) at the site of the tibial tuberosity.
Presentation
Classically found in sporty adolescent boys. Causes:
-
Usually mild, intermittent pain in the lower front of the knee, particularly over the tibial tuberosity
-
Pain worse during activities like running, jumping and going up/down stairs
-
Pain can be reproduced with extension against resistance
-
Can lead to an avulsion fracture, where the tuberosity is separated from the rest of the tibia.
Management
Risk can be reduced by keeping the hamstrings and quadriceps flexible (with stretches).
Treatment is generally RICE (rest, ice, compression, elevation), simple pain medication, and physiotherapy after the initial symptoms have improved.
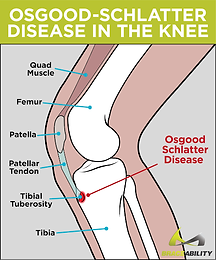
Different types of pain and common disorders causing pain **
